Fundamentals of Asymmetric Catalysis
Patrick J. Walsh University of Pennsylvania
Marisa C. Kozlowski University of Pennsylvania
Preface
Introduction to Fundamentals of Asymmetric Catalysis
The importance of stereochemistry 1 in chemical interactions is crucial in several fields of chemistry including pharmaceutical, biological, agricultural, fragrance, and materials chemistries. This is probably best appreciated in the context of drug-receptor interactions, because most biological targets are chiral entities. Hence, there is enormous demand to devise viable and practical methods for preparing chiral compounds as single enantiomers. It is this demand that drives the field of asymmetric synthesis.
An integral part of asymmetric synthesis is asymmetric catalysis, which is dedicated to the development and applications enantioenriched catalysts to transform prochiral and racemic substances into valuable enantioenriched synthetic building blocks. Since the first reports appeared in the 1960s, a wide variety of chiral organometallic complexes and organocatalysts (catalysts without a reactive metal component) have been identified as asymmetric catalysts. 2-8 These catalysts not only effect useful reactions with high levels of enantioselectivity, but often do so with a broad range of substrates. 9 The Royal Swedish Academy of Sciences awarded the 2001 Nobel Prize in Chemistry to pioneering figures in the field of asymmetric catalysis, K. Barry Sharpless, William S. Knowles, and Ryoji Noyori, in recognition of these achievements: for “the development of catalytic asymmetric synthesis.” In the following chapters, we outline the fundamental principles of asymmetric catalysis, a rapidly evolving field on the cutting edge of organic synthesis and organometallic chemistry.
Goals and Intended Audience
This text was written to introduce the fundamental concepts of asymmetric catalysis to chemists with some graduate level education or to advanced undergraduate students. It provides a treatment beyond the introductory level. This book is intended to be a useful source of information to professionals working in organic synthesis who have occasion to employ enantioselective catalysts and desire to increase their level of understanding and chances of a successful outcome.
Organization of this Book
This book is organized differently than most books on asymmetric catalysis. Rather than classifying by reaction type, the chapters are organized by concepts. Although the choice of reactions and catalysts to include was primarily based on their educational significance, examples have been selected so that most of the commonly encountered reactions, ligands, and catalysts in asymmetric catalysis are represented. With an emphasis on instructional value, the coverage of historical significance and the latest developments in catalyst optimization for a given reaction have been included in the references and can be found in more specialized and/or comprehensive collections and reviews. For comprehensive presentation by reaction type, the reader is directed to other excellent texts. 4,6,8
Each chapter can be read separately and provides the underlying concepts along with selected examples. A detailed appendix defines the key terms, types of chirality, and nomenclature of asymmetric transformations (prochiral etc.) and is intended as a reference source.
Some of the mechanisms discussed herein are mechanistic proposals and are presented as a framework for understanding the material and discussion. Further exploration of many of these mechanisms is expected and there will undoubtedly be changes as the understanding of the community evolves.
In this book, enantiomeric excess (ee) is used rather than enantiomeric ratios (er) as this in the current state found in most publications. Nonetheless, a compelling argument for the use of enantiomeric ratios can be made. 10 Before this can occur, a convention must be established as to how the enantiomeric ratios are reported (x:1 vs a fraction summing to 100%, i.e. 95:5). We support the latter since it is the most readily correlated to the difference in energy accounting for kinetic control of the product ratios in asymmetric reactions (see Equation A.2 and Equation 1.1).
Challenges in Asymmetric Catalysis
Despite significant effort, the state of the art still falls short in many ways. The ideal catalytic asymmetric transformation would proceed in 100% yield, and would provide complete chemo-, regio-, and stereocontrol (diastereo- and enantioselectivity). It would be conducted with a minimum of solvent and additives, generate no wasteful byproducts, and employ an inexpensive recoverable catalyst at low loadings. For large scale processes, the turnover number (TON) and turnover frequency (TOF) are particularly important. The TON describes the catalyst loading as the number of reaction cycles each catalyst molecule completes. The TOF is the number of reaction cycles per unit time. The latter is particularly important given the high cost per hour of operating large scale chemical reactors. Few catalytic asymmetric transformations meet these rigorous criteria. As a result, many industrial processes for the synthesis of enantiomerically pure materials still rely on classical resolution of racemic mixtures or employ enzymatic resolution. The most progress toward the above goals has been made with catalytic asymmetric reductions and oxidations. 11-13 Illustrative examples include the asymmetric hydrogenation of a precursor en route to (L)-DOPA, 14,15 an anti-Parkinson’s disease agent, and the asymmetric epoxidation of allyl alcohol to produce enantioenriched glycidol, an important chiral building block 16 (see Figure). While some catalytic asymmetric carbon-carbon bond forming transformations 13 approach the above criteria, as is the case with hydroformylation 17,18 or p–allylation, 19 the economics for utilizing these processes for large scale production are not yet favorable. An example of one of the few production scale asymmetric carbon-carbon bond forming reactions is the asymmetric cyclopropanation of 2-methylpropene to yield an intermediate for cilastatin, 20,21 an agent that is coadminstered with the antibiotic imipenem to prevent its degradation by renal dehydropeptidase. In this book, the fundamentals of asymmetric catalysis are examined to provide a basis for the further development of practical asymmetric catalysts that can meet the above criteria.
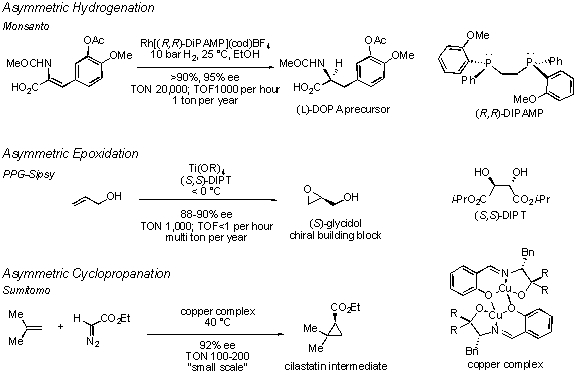
Examples of highly efficient catalytic asymmetric reactions that are performed on large scale.
Topics Covered
Chapter 1 introduces the major types of asymmetric transformations (asymmetric catalysis with prochiral substrates, kinetic resolution and dynamic kinetic resolution of racemates) along with their corresponding energy diagrams. The relationship between energy and asymmetric induction is described. The effects of competing background processes are also discussed in terms of energy diagrams. Chapter 2 (Lewis acid and Lewis base) and Chapter 3 (Brønsted acid, Brønsted base, ion-pairing, group transfer, cross-coupling, and p-activation) outline most of the chemical activation modes found in asymmetric catalysts. Many catalysts combine these activation features leading to bifunctional, dual, and even multifunctional catalysts (Chapter 12). The transfer of stereochemical information from the catalyst to the reacting substrates is discussed in Chapter 4 (classical interactions such as steric occlusion) and Chapter 5 (non-classical interactions such as cation-p). Methods for chiral catalyst optimization by means of additives (chiral activation, chiral deactivation, and modification with achiral ligands) are surveyed in Chapter 6. Chapters 7-9 describe the various forms of resolution: kinetic resolution, parallel kinetic resolution, dynamic kinetic resolution, and dynamic kinetic asymmetric transformations. As shown in Chapter 11, impure chiral catalysts can be highly advantageous (nonlinear effects) and are key to explaining the evolution of enantiopurity in nature (autoinduction and autocatalysis). Chapter 15 surveys the different types of supported chiral catalysts that combine the best features of homogeneous and heterogeneous catalysts.
Most practical asymmetric catalysts have focused on the generation of small chiral molecules containing one to two stereogenic units in highly enantioenriched form. Such compounds can be used as the starting point in the synthesis where all further stereogenic units are introduced diastereoselectively, thereby maximizing the contribution of the often valuable chiral component. The development of tandem or cascade processes (Chapter 14) that combine these events allows the rapid synthesis of highly complex structures. A different tactic is the use of desymmetrization (Chapter 10) to rapidly generate complex stereochemical arrays. The concept of using enantioenriched catalysts not only to create smaller subunits but also to assemble chiral subunits in transformations that generate additional stereogenic units has not received widespread acceptance. However, such double diastereoselective or triple diastereoselective processes hold much potential (Chapter 13). If inexpensive catalysts that exercise complete stereocontrol were available for every possible diastereomeric combination for a given process, then the assembly of complex stereochemical arrays would be highly simplified and could be practiced even by non-experts.
Alternatively, the convergent assembly of higher order structures with multiple stereogenic units can be undertaken from several different small chiral components (Chapter 16). By using enantiopure components, a convergent synthesis can provide a flexible approach to most if not all of the diastereomeric congeners. This approach has been the most successful when the convergent assembly transforms do not create further stereogenic units thereby removing the need for diastereoselectivity optimization. Another area that has received less attention is the use of chiral catalysis in transformations of complex or high value substrates. Most often chiral catalysts are employed to generate small chiral structures and can often do so with a high degree of substrate generality. The use of chiral catalysts with complex substrates containing multiple functional groups, however, is less explored (Chapter 16). Here, selective functionalization is crucial. Only chiral catalysts that are highly reliable can be employed in such situations where the substrates are valuable. Clearly, there are still many challenges facing the field of asymmetric catalyst development.
As a prerequisite to any useful discussion of asymmetric catalysis, a common means of describing various asymmetric groups and chirality types is required. In the Appendix, the definitions of chiral substances, their precursors, and their nomenclature are outlined. Different types of chiral compounds possessing a variety of stereogenic units, including central, axial, and planar chirality, are outlined. Achiral precursors that are prochiral, meaning that they can be transformed into enantiomerically pure chiral compounds, are delineated. The generation of pure enantiomers from racemic mixtures is also presented. Examples of the creation of each type of enantiomerically pure stereogenic unit are outlined. The Appendix concludes with strategies for the creation of multiple stereogenic units in a controlled manner to generate single enantiomeric and diastereomeric compounds.
Asymmetric catalysis is a challenging but exciting area of endeavor. The ability to control the generation of nearly identical chemical compounds has caught the imagination of many outstanding scientists. In some ways, the field is beginning to mature (nearly 50 years old!). Even so, the stimulating discoveries within the last few years reveal the incredible potential in this field. There are many challenges remaining and, undoubtedly, many ingenious methods will be devised to address them. We hope that the community finds this text useful in the pursuit of this endeavor. We would like to thank the many individuals who have provided inspiration to us in the writing of this book. We are especially grateful to those who have read and commented on the text allowing it to reach its current form. In particular, Mukund Sibi (North Dakota State University) who read the entire text and Jennifer Love (University of British Columbia), Mathew Sigman (University of Utah), Jeffrey Johnson (University of North Caroline, Chapel Hill) who read large sections. Additional experts were consulted for individual chapters, including John Brown (Oxford University), David Glueck (Dartmouth College), Chris Vanderwal (University of California, Irvine), Huw Davies (SUNY Buffalo), Greg Cook (North Dakota State University) and Tomislav Rovis (Colorado State University). Their suggestions were valuable and insightful.
Patrick J. Walsh
Marisa C. Kozlowski
University of Pennsylvania
References
(1) Eliel, E. L.; Wilen, S. H.; Mander, L. N. Stereochemistry of Organic Compounds; Wiley-Interscience: New York, 1994.
(2) Asymmetric Synthesis; Aitken, R. A.; Kilényi, S. N., Eds.; Chapman & Hall: London, 1992.
(3) Koskinen, A. Asymmetric Synthesis of Natural Products; John Wiley & Sons: Chichester, 1993.
(4) Catalytic Asymmetric Synthesis; Ojima, I., Ed.; VCH: New York, 1993.
(5) Noyori, R. Asymmetric Catalysis in Organic Synthesis; Wiley-Interscience: New York, 1994.
(6) Nógrádi, M. Stereoselective Synthesis; VCH: Weinheim, 1995.
(7) Gawley, R. E.; Aube, J. Principles of Asymmetric Synthesis; Pergamon: Oxford, 1996; Vol. 14.
(8) Comprehensive Asymmetric Catalysis; Jacobsen, E. N.; Pfaltz, A.; Yamamoto, H., Eds.; Springer Verlag: New York, 1999; Vol. 1-3.
(9) Yoon, T. P.; Jacobsen, E. N. “Privileged Chiral Catalysts” Science 2003, 299, 1691-1693.
(10) Gawley, R. E. “Do the Terms “% ee” and “% de” Make Sense as Expressions of Stereoisomer Composition or Stereoselectivity?” J. Org. Chem. 2006, 71, 2411-2416.
(11) Blaser, H. U.; Spindler, F.; Studer, M. “Enantioselective Catalysis in Fine Chemicals Production” Applied Catalysis A: General 2001, 221, 119–143.
(12) Asymmetric Catalysis on Industrial Scale: Challenges, Approaches and Solutions; Blaser, H. U.; Schmidt, E., Eds.; Wiley: New York, 2004.
(13) Farina, V.; Reeves, J. T.; Senanayake, C. H.; Song, J. J. “Asymmetric Synthesis of Active Pharmaceutical Ingredients” Chem. Rev. 2006, 106.
(14) Vineyard, B. D.; Knowles, W. S.; Sabacky, M. J.; Bachman, G. L.; Weinkauff, D. J. “Asymmetric Hydrogenation. Rhodium Chiral Bisphosphine Catalyst” J. Am. Chem. Soc. 1977, 99, 5946-5952.
(15) Knowles, W. S. “Asymmetric Hydrogenation” Acc. Chem. Res. 1983, 16, 106-112.
(16) Shum, W.; Cannarsa, M. In Chirality in Industry II: Developments in the Commercial Manufacture and Applications of Optically Active Compounds; Collins, A. N., Sheldrake, G., Crosby, J., Eds.; Wiley: New York, 1997; pp 363-.
(17) Chapuis, C.; Jacoby, D. “Catalysis in the Preparation of Fragrances and Flavours” Applied Catalysis A: General 2001, 221, 93–117.
(18) Breit, B.; Seiche, W. “Recent Advances on Chem-, Regio-, and Stereoselective Hydroformylation” Synthesis 2001, 1-36.
(19) Trost, B. M.; Crawley, M. L. “Asymmetric Transition-Metal-Catalyzed Allylic Alkylations: Applications in Total Synthesis” Chem. Rev. 2003, 103, 2921-2943.
(20) Aratani, T. “Catalytic Asymmetric-Synthesis of Cyclopropane-Carboxylic Acids – an Application of Chiral Copper Carbenoid Reaction” Pure Appl. Chem. 1985, 57, 1839-1844.
(21) Aratani, T. In Comprehensive Asymmetric Catalysis; Jacobsen, E. N., Pfaltz, A., Yamamoto, H., Eds.; Springer Verlag: New York, 1999; Vol. 3; pp 1451-1460.